Applied NAPL Science Review
Natural Source Zone Depletion Screening Methodologies
Editor: Lisa Reyenga, PE
ANSR Scientific Advisory Board
J. Michael Hawthorne, PG, Board Chairman, GEI Consultants, Inc
Andrew J. Kirkman, PE, BP Corporation North America
Robert Frank, RG, Jacobs
Paul Cho, PG, CA Regional Water Quality Control Board-LA
Randy St. Germain, Dakota Technologies, Inc.
Dr. Terrence Johnson, USEPA
Stephen S. Boynton, PE, LSP, Subsurface Env. Solutions, LLC
Dr. Randall Charbeneau, PE, University of Texas
Mark Lyverse, PG, Chevron Energy Technology Company
Brent Stafford, Shell Oil Co.
Douglas Blue, Ph.D., ExxonMobil Environmental & Property Solutions
Applied NAPL Science Review (ANSR) is a scientific ejournal that provides insight into the science behind the characterization and remediation of Non-Aqueous Phase Liquids (NAPLs) using plain English. We welcome feedback, suggestions for future topics, questions, and recommended links to NAPL resources. All submittals should be sent to the editor.
DISCLAIMER: This article was prepared by the author(s) in their personal capacity. The opinions expressed in this article are the author’s own and do not necessarily reflect the views of Applied NAPL Science Review (ANSR) or of the ANSR Review Board members.
Natural Source Zone Depletion Screening Methodologies
GEI Consultants, Inc.
BP Corporation North America
Qualitative or semi-quantitative evaluation of NSZD has been used to evaluate if NSZD processes are ongoing and is termed “NSZD Screening” (Jewell and Wilson 2011). It is typically easier to implement and less costly than quantitative methods to estimate NSZD rates.
Examples of where NSZD Screening can be used for LNAPL sites include:
- Supporting site closure where site-specific metrics (e.g. maximum extent practical for LNAPL recovery) have been met and understanding of the fate of the source is desired.
- Refining the CSM to support a remedy evaluation. For example, providing an improved understanding of subsurface mechanisms to support enhanced bioremediation performance estimates and to determine or design the applicable methodologies for quantitative NSZD rate estimates.
- Contributing to an ongoing monitoring program to monitor progress toward site-specific metrics. NSZD Screening confirms that NSZD processes are continuing over time while compliance monitoring of specific exposure routes is performed separately.
NSZD Screening methodologies include, but are not limited to:
- Soil Gas Screening;
- Groundwater Screening;
- Thermal Screening; and
- Screening for LNAPL Compositional Change.
Each methodology is introduced below and the relative advantages and limitations are summarized in Table 1.
Soil Gas Screening
Soil gas screening involves collecting soil gas readings for oxygen, methane, and carbon dioxide. Typically, the data can be collected with a field gas meter from pre-existing vapor monitoring points or groundwater monitoring wells. Evidence that NSZD is ongoing includes depleted oxygen and enriched carbon dioxide and/or methane concentrations in comparison to background (Jewell and Wilson 2011, Sweeney and Ririe 2017). It is necessary to use an activated carbon or charcoal filter when field screening for methane to reduce interference from volatile organic compounds.
Figure 1 – In soil gas screening NSZD is indicated by decreased concentrations of oxygen and increased concentrations of carbon dioxide and/or methane, relative to background.
Groundwater Screening
There are two approaches for NSZD Screening in groundwater. The most familiar is the use of geochemical parameters in groundwater. This method is primarily applicable to NSZD occurring through the dissolution pathway and is referred to as natural attenuation (NA) or the natural attenuation indicating parameters (NAIPs) method (CRC Care 2018, ITRC 2018). NA is a component of NSZD, so evidence of NA indicates that NSZD is ongoing.
Another groundwater screening option is the degassing method. Dissolved gases (e.g. nitrogen, argon) can be “stripped” from groundwater due to partitioning into gaseous methane bubbles produced as a product of methanogenic biodegradation. Evidence of depleted dissolved gases and enriched dissolved methane in groundwater indicates that NSZD is ongoing (Amos et al 2005).
Figure 2 -Dissolved inert gases partition into gaseous methane bubbles biogenically produced in groundwater. NSZD is indicated by a linear relationship between methane concentration and nitrogen depletion relative to background (modified from Amos et al 2005).
Thermal Screening
Thermal screening involves collecting a vertical profile of soil temperature. Typically, the data can be collected with inexpensive equipment (e.g. iButtons or thermistors) from pre-existing wells. An increased temperature in the LNAPL source area in comparison to background indicates that NSZD is ongoing (Sweeney and Ririe 2014, API 2017, CRC Care 2018, ITRC 2018).
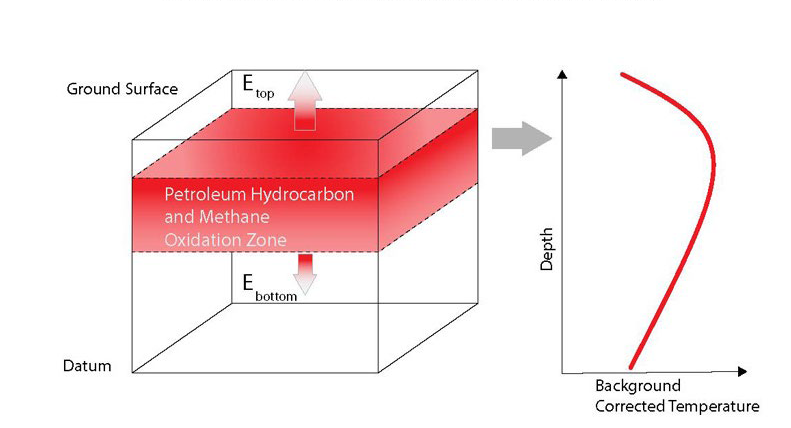
Screening for LNAPL Compositional Change
LNAPL samples are collected and analyzed in a laboratory via gas chromatography–mass spectrometry (GC/MS) or gas chromatography with flame-ionization detection (GC/FID) to screen for LNAPL compositional change. The depletion of soluble and volatile constituents (“weathering”) in the chromatograms indicates that NSZD is ongoing (CRC Care 2018).
Table 1 – Summary of the advantages and limitations of each screening methodology.
A Word of Caution
A thorough understanding of the CSM and the regulatory environment is required to effectively implement NSZD Screening at a site. Screening data are useful to document that NSZD processes are ongoing, but NSZD Screening does not directly provide quantitative NSZD rates. Critical aspects of the CSM to interpret qualitative and quantitative results include soil profile and moisture content relative to soil gas diffusion behavior. Source delineation vertically and laterally combined with water table information will identify where to direct measurements vertically and spatially. The LNAPL composition will aide in understanding existing composition as well as dominant weathering mechanisms, such as biodegradation versus volatilization combined with biodegradation. Whether NSZD alone is sufficient to protect both health and the environment is dependent on a comprehensive CSM and risk assessment.
References:
Amos, R.T., K.U. Mayer, B.A. Bekins, G.N. Delin, and R.L. Williams, 2005. Use of dissolved and vapor-phase gases to investigate methanogenic degradation of petroleum hydrocarbon contamination in the subsurface. Water Resources Research, 41(2), doi:10.1029/2004WR003433.
API, 2017. Quantification of Vapor Phase-related Natural Source Zone Depletion Processes. API Publication 4784, First Edition, May 2017. American Petroleum Institute, 124 pp. https://www.techstreet.com/standards/api-publ-4784?product_id=1984357
CRC Care, 2018, Technical measurement guidance for LNAPL natural source zone depletion, Cooperative Research Centre for Contamination Assessment and Remediation of the Environment, Technical Report series, no. 44 August 2018. https://www.crccare.com/publications/technical-reports
Garg, Sanjay, Charles J. Newell, Poonam R. Kulkarni, David C. King, David T. Adamson, Maria Irianni Renno, and Tom Sale, 2017. Overview of Natural Source Zone Depletion: Processes, Controlling Factors, and Composition Change. Groundwater Monitoring & Remediation 37, no. 3/ Summer 2017/pages 62–81.
ITRC, 2018. Light Non-Aqueous Phase Liquids (LNAPL) Document Update: Evaluating and Implementing LNAPL Remedial Technologies. Interstate Technology Regulatory Council, LNAPL Update Team, pending publication March 2018. https://www.itrcweb.org/Team/Public?teamID=73
Jewell, Kenneth P. and John T. Wilson, 2011. A New Screening Method for Methane in Soil Gas Using Existing Groundwater Monitoring Wells. Groundwater Monitoring and Remediation. Vol 31, no 3, Summer 2011, pg 82-94.
Ng, C.G.H., B.A. Bekins, I.M. Cozarelli, M.J. Baedecker, P.C. Bennett, R.T. Amos, and W.N. Herkelrath, 2015. Reactive transport modeling of geochemical controls on secondary water quality impacts at a crude oil spill site near Bemidji, MN, Water Resources Research. DOI:10.1002/2015WR016964
Sweeney, Robert E. and George Todd Ririe, 2014. Temperature as a Tool to Evaluate Aerobic Biodegradation in Hydrocarbon Contaminated Soil. Groundwater Monitoring and Remediation. Vol 34, no 3, Summer 2014, pg 41-50.
Sweeney, Robert E. and George Todd Ririe, 2017. Small Purge Method to Sample Vapor from Groundwater Monitoring Wells Screened Across the Water Table. Groundwater Monitoring and Remediation. Vol 37, no 4, Fall 2017, pg 51-59.
Research Corner
Thank you to Dr. Tom Sale of the Colorado State University, Center for Contaminant Hydrology, for providing access to selected graduate level NAPL research.
Biotic control of LNAPL longevity – laboratory and field- scale studies
Eric Douglas Emerson
Master of Science
Colorado State University
Abstract:
Natural source zone depletion (NSZD) is an emerging strategy for managing light nonaqueous phase liquids (LNAPLs). Unfortunately, little is known about NSZD rates over extended periods of time, where heterogeneous redox conditions and changing LNAPL saturations may influence processes governing losses. Understanding long-term rates is central to anticipating LNAPL longevity under both natural and engineered conditions. Herein, laboratory and field-scale modeling studies were conducted to evaluate LNAPL longevity. Laboratory studies evaluated loss rates as a function of total contaminant concentration under sulfate-reducing (SR) and methanogenic (MG) conditions. Biotic and abiotic loss rates were determined via tracking biodegradation products and hydrocarbons in column effluents and produced gasses over time. Furthermore, compositional weathering of LNAPL was evaluated. Loss rates with elevated sulfate averaged 39.8 mmole carbon/day/m3 (±9.1 mmole carbon/day/m3). Once sulfate in the soil was depleted to influent water sulfate concentrations of 20 mg/L, subsequent average loss rates were 39.7 mmole carbon/day/m3 (±19.6 mmole carbon/day/m3). Overall, loss rates with and without elevated sulfate were similar. Furthermore, results suggested that loss rates are independent of LNAPL concentration over the range of 9,000 to 37,000 mg/kg and redox conditions observed. Loss rates independent of LNAPL concentrations indicated that biologically mediated NSZD follows zero-order kinetics over the range of conditions evaluated. Column loss rates were compared to field-measured loss rates assuming an LNAPL thickness of three meters. Given this assumption, mean observed early- and late-loss rates are 1.38 and 1.41 μmole carbon/m2/sec, respectively. Assuming decane as a representative LNAPL, observed loss rates are equivalent to 7890 and 8060 L/hectare/year. A column was sacrificed at the completion of the study. Predicted mass losses of the study equate to approximately 1% total initial LNAPL mass lost. Total petroleum hydrocarbons (TPH) soil analysis of initial and final grab samples of column soil did not detect significant mass losses. Moreover, no significant shifts in the LNAPL composition were seen during the course of the study. Mass losses in this range are difficult to accurately quantify via soil-phase hydrocarbon analyses, thus highlighting the utility of the approach used herein. An LNAPL longevity model (The Glide Path Model) was applied at a field site using a zero-order rate model for biological NSZD. LNAPL Longevity ranged from 35 to 105 years using a mean NSZD rate, plus or minus factors of 2 and ½, respectively. Active recovery was shown to have little effect on the longevity of LNAPL.
The primary objective of ANSR is the dissemination of technical information on the science behind the characterization and remediation of Light and Dense Non-Aqueous Phase Liquids (NAPLs). Expanding on this goal, the Research Corner has been established to provide research information on advances in NAPL science from academia and similar research institutions. Each issue will provide a brief synopsis of a research topic and link to the thesis/dissertation/report, wherever available.
Related Links
API LNAPL Resources
ASTM LCSM Guide
Env Canada Oil Properties DB
EPA NAPL Guidance
ITRC LNAPL Resources
ITRC LNAPL Training
ITRC DNAPL Documents
RTDF NAPL Training
RTDF NAPL Publications
USGS LNAPL Facts
ANSR Archives
Coming Up
In coming newsletters, look for more articles on natural source zone depletion as well as NAPL mobility in sediment, surface water sheen discharge management, lifecycle NAPL management, and applications for data automation at NAPL sites.
Announcements
Upcoming Training – Learn More Here: https://www.itrcweb.org/Training
- July 14: PFAS Roundtable (Part 1 of the Series)
- August 20: Integrated DNAPL Site Characterization
- August 25: Characterization and Remediation in Fractured Rock
- August 27: Optimizing Injection Strategies and In Situ Remediation Performance
- September 3: Long-term Contaminant Management Using Institutional Controls
- September 15: Connecting the Science to Managing LNAPL Sites Part 1: Understanding LNAPL Behavior in the Subsurface
- September 17: TPH Risk Evaluation at Petroleum-Contaminated Sites
- September 22: Connecting the Science to Managing LNAPL Sites Part 2: LNAPL Conceptual Site Models and the LNAPL Decision Process
- September 29: Connecting the Science to Managing LNAPL Sites Part 3: Using LNAPL Science, the LCSM, and LNAPL Goals to Select an LNAPL Remedial Technology
Upcoming Conference Abstract Deadlines
- Battelle 2021 Sediments Conference (January 25-28, 2021) Abstracts Due June 30, 2020
- AEHS 30th Annual International Conference on Soil, Water, Energy, and Air (March 22-25, 2021) Abstracts due July 1, 2020
- Battelle 2021 Combined Chlorinated and Bioremediation Conference (June 27 – July 1, 2021) Abstracts due August 31, 2020
ANSR now has a companion group on LinkedIn that is open to all and is intended to provide a forum for the exchange of questions and information about NAPL science. You are all invited to join by clicking here OR search for “ANSR – Applied NAPL Science Review” on LinkedIn. If you have a question or want to share information on applied NAPL science, then the ANSR LinkedIn group is an excellent forum to reach out to others internationally.