J. Michael Hawthorne, PG, Board Chairman, GEI Consultants, Inc.
Andrew J. Kirkman, PE, BP Corporation North America
Robert Frank, RG, Jacobs
Paul Cho, PG, CA Regional Water Quality Control Board-LA
Randy St. Germain, Dakota Technologies, Inc.
Dr. Terrence Johnson, USEPA
Brent Stafford, Shell Oil Co.
Douglas Blue, Ph.D., Imperial Oil Environmental & Property Solutions (Retired)
Natasha Sihota, Ph.D., Chevron
Kyle Waldron, Marathon Petroleum
Danny D. Reible, Professor at Texas Tech University
Reeti Doshi, National Grid
Frequently Asked Questions
DISCLAIMER: This article was prepared by the author(s) in their personal capacity. The opinions expressed in this article are the author’s own and do not necessarily reflect the views of Applied NAPL Science Review (ANSR) or of the ANSR Review Board members.
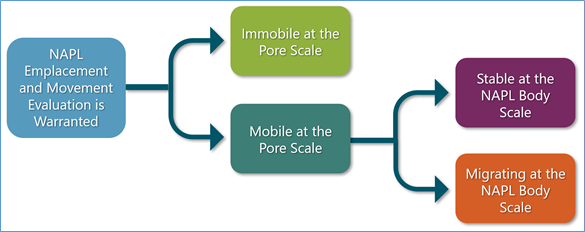
- If the NAPL movement occurs only within the NAPL body, then the NAPL is stable; in this case, the NAPL does not move at the NAPL body scale and will not advectively expand in any direction under observed or reasonably anticipated field conditions.
- If the NAPL movement includes entry into pore spaces beyond the NAPL Body extent, then the NAPL body is migrating; in this case, the NAPL body may advectively expand in at least one direction under observed or reasonable anticipated field conditions.
To help evaluate whether a NAPL body may migrate to a degree that could pose a risk to receptors, multiple lines of evidence (LOE’s) can be used. An example WOE method (adapted from ASTM E3282-21a) that can be used to help make this interpretation is discussed below.
Example WOE Approach to Interpret NAPL Mobility Test Results
Table 1 presents an example of a WOE approach to interpret whether a NAPL body may migrate to receptors under observed or reasonably anticipated field conditions. The evaluation focuses on the potential for upward NAPL migration, because in sediment systems, the potential for exposure to receptors increases near the sediment surface.
The approach includes four LOEs and a scoring method for each LOE. The LOE’s and the rationale behind the scoring method are presented in Table 1 (adapted from ASTM E3282-21).
Line of Evidence | Scoring | Scoring Rationale |
---|---|---|
1. NAPL vertical gradient is net downward | +1 if net vertical gradient is downward most of the time 0 if net vertical gradient direction is variable or uncertain -1 if net vertical gradient is upward most of the time | If net vertical gradient direction is downward most of the time, the NAPL may migrate downward (away from the most common potential receptors), and upward migration is unlikely. If net vertical gradient direction is upward most of the time, the NAPL may migrate upward (toward potential surface receptors). |
2. Calculated critical NAPL body thickness | +1 if NAPL body thickness is less than calculated critical thickness 0 if NAPL body thickness is uncertain -1 if NAPL body thickness is more than calculated critical thickness | In order to have enough NAPL capillary pressure at the top of the NAPL body to exceed the pore entry pressure of the overlying sediment, the NAPL body thickness must exceed a critical value. If the NAPL thickness is less than this value, upward NAPL migration is not possible. |
3. NAPL travel distance prior to depletion | +1 if NAPL saturation would be reduced to immobile saturation before reaching receptor (for example, surface sediment) -1 if NAPL saturation would not be reduced to immobile saturation before reaching receptor | If the NAPL is depleted to immobile saturation before encountering a receptor, it will not be able to continue migrating toward that receptor. If the calculation shows otherwise, it may be possible for the NAPL to migrate far enough to reach the receptor. |
4. Calculated NAPL pore velocity | +1 if <10-7 cm/s -1 if >10-7 cm/s | This threshold is site-specific and depends on the distance to the nearest sensitive receptor. In most situations, NAPL pore velocities of <10-7 cm/s are considered to represent de minimis NAPL migration. For situations where the NAPL is in close proximity to the receptor, a lower threshold may need to be chosen. |
It should be noted that a score of +1 for any one of these LOEs could actually be considered sufficient evidence to conclude that the NAPL cannot migrate to a receptor in a timeframe that may pose a concern. However, using a WOE approach is more conservative, and increases protectiveness and confidence in the results
Discussion
Applying this WOE NAPL body migration evaluation approach is relatively straightforward:
- score each LOE
- sum the scores
- interpret the results
Additional information regarding each LOE is provided below. The reader should refer to ASTM E3282-21a for details regarding the calculation methods and data required to evaluate each LOE.
LOE 1 is a calculation of the net vertical gradient acting on the NAPL due to two gradient components: 1) the vertical hydraulic gradient associated with groundwater flow; and 2) the hydraulic gradient due to gravity, which is caused by the density contrast between the NAPL and the surrounding groundwater. If the sum of these driving forces is net downward, then the NAPL cannot migrate upward.
LOE 2 considers the pore entry pressure of the material above the NAPL body. Upward mobility of non-wetting NAPL that the capillary pressure overcome the pore entry pressure of the overlying porous medium. If the actual vertical thickness of contiguous mobile NAPL in sediment is less than the calculated critical vertical thickness required to exceed the pore entry pressure, then non-wetting NAPL will not migrate into the overlying capillary barrier.
LOE 3 accounts for the depletion of NAPL from a NAPL body, if migrating. When disconnected from its original source, a migrating NAPL body leaves NAPL behind it at immobile saturation. Therefore, the overall NAPL saturation decreases as the NAPL body migrates. Eventually, the NAPL body is no longer capable of migrating (that is, it is stable). If this would occur before the NAPL could reach a receptor, the NAPL will not be able to migrate to that receptor.
LOE 4 considers the theoretical NAPL velocity within the pore spaces, under the assumption that the NAPL body is migrating. If the calculated NAPL velocity toward a potential receptor (for example, the sediment surface), is below a de minimis threshold value (the magnitude of this threshold value depends on the distance to the receptor), the NAPL body is considered stable for practical purposes. For weathered, viscous NAPLs in fine-grained sediment, calculated pore velocities often are so low that the NAPL can be considered immobile for practical purposes. This criterion is inherently conservative because continued NAPL weathering and dissolution will continue to:
- reduce NAPL saturations;
- preferentially deplete NAPL of lighter, more soluble compounds;
- increase NAPL density; and
- increase NAPL viscosity.
All of these factors will further decrease any potential for upward NAPL movement over time
Example Application of WOE Evaluation Method
During a NAPL mobility evaluation, NAPL was encountered within an approximately 15-cm (0.5-ft) thick sediment interval, 305-320 cm (10-10.5 ft) below the mudline.
To support LOE 1 and LOE 2, a broad range of potential NAPL physical parameters from the literature was used, in combination with location-specific sediment physical parameters and hydraulic parameters. LOE 3 was evaluated using NAPL saturation values measured in the 15-cm (0.5-ft) thick interval of interest and within the overlying sediment, and a range of estimated NAPL immobile saturation values. LOE 4 was evaluated using initial NAPL saturation and NAPL effective hydraulic conductivity based on laboratory NAPL mobility test results, and location-specific sediment physical parameters and hydraulic parameters.
Based on these results, the WOE score was evaluated as follows.
- LOE 1: Net vertical gradient uncertain; based on literature-based NAPL density values, the predominant net gradient could be upward or downward à LOE score = 0
- LOE 2: Critical NAPL body thickness required to exceed pore entry pressure at top of NAPL body calculated as at least 79 cm (2.6 ft), which is greater than the observed NAPL thickness of 15 cm (0.5 ft) à LOE score = +1
- LOE 3: Calculated NAPL travel distance prior to depletion to immobile saturation ranged from >6 to 15 cm (0.2 to 0.5 ft), which is considerably less than the thickness of overlying sediment (305 cm, or 10.0 ft) à LOE score = +1
- LOE 4: The estimated NAPL pore velocity, if migrating, was calculated as 1.5×10-7 cm/sec (0.15 ft/yr) à LOE score = -1
- Sum of LOE scores = +1
Because the WOE score is positive (>0), the NAPL is considered to be stable at the NAPL body scale under observed or reasonably anticipated field conditions by the WOE evaluation. In other words, it is unlikely that the NAPL can migrate upward to surface receptors and pose a concern or risk
Conclusions
The example WOE process provides an objective methodology to characterize whether a NAPL body may migrate to a potential receptor under observed or reasonably anticipated field conditions. Using objective criteria can get multi-stakeholder groups all on the same page. Using multiple LOEs and evaluating them holistically in a WOE process enhances confidence in the resulting interpretations regarding potential NAPL body migration.
A Word of Caution
To develop a robust assessment of NAPL movement at a given site, it is prudent to consider a range of potential values for any estimated parameters, and conduct sensitivity analyses.
References
ASTM E3268−20. 2020. “Standard Guide for NAPL Mobility and Migration in Sediment – Sample Collection, Field Screening, and Sample Handling.”
ASTM E3282−21a. 2021. “Standard Guide for NAPL Mobility and Migration in Sediments – Evaluation Metrics.”
Gefell, M. J., 2021. A Weight-of-Evidence Approach to Characterize Pore-Scale NAPL Mobility in Sediment Samples”. Applied NAPL Science Review, Vol. 9, Issue 8, December 2021.
Reyenga, L., 2021a. “Evaluating Emplacement and Movement of NAPL in Sediment”. Applied NAPL Science Review, Vol. 9, Issue 1, February 2021.
Reyenga, L., 2021b. “Typical Metrics for Evaluating Advective NAPL Movement in Sediments”.
Vol. 9, Issue 5, August 2021.
Research Corner

Doctor of Philosophy
Colorado State University
Abstract
A series of controlled experiments were conducted to develop the C3 tools and methods. The critical aspects of C3 are downhole circulation of liquid nitrogen via a cooling system, the strategic use of thermal insulation to focus cooling into the core, and the use of back pressure to optimize cooling. The C3 methods were applied at two contaminated sites: 1) F.E. Warren (FEW) Air Force Base near Cheyenne, WY and 2) a former refinery in the western U.S. The results indicated that the rate of core collection using the C3 methods is on the order of 30 foot/day. The C3 methods also improve core recovery and limits potential biases associated with flowing sands.
HTA of frozen core was employed at the former refinery and FEW. Porosity and fluid saturations (i.e., aqueous, non-aqueous liquid, and gas) from the former refinery indicate that given in situ freezing, the results are not biased by drainage of pore fluids from the core during sample collection. At FEW, a comparison between the results of HTA of the frozen core collected in 2014 and the results of site characterization using unfrozen core, (second-generation (2G) methods) at the same locations (performed in 2010) indicate consistently higher contaminant concentrations using C3. Many factors contribute to the higher quantification of contaminant concentrations using C3. The most significant factor is the preservation of the sediment attributes, in particular, pore fluids and volatile organic compounds (VOCs) in comparison to the unfrozen conventional sediment core.
The NMR study was performed on laboratory-fabricated sediment core to resolve NAPL distribution within the porous media qualitatively and quantitatively. The fabricated core consisted of Colorado silica sand saturated with deionized water and trichloroethylene (TCE). The cores were scanned with a BRUKER small-animal scanner (2.3 Tesla, 100 MHz) at 20 °C and while the core was frozen at -25 °C. The acquired images indicated that freezing the water within the core suppressed the NMR signals of water-bound hydrogen. The hydrogen associated with TCE was still detectable since the TCE was in its liquid state (melting point of TCE is -73 °C). Therefore, qualitative detection of TCE within the sediment core was performed via the NMR scanning by freezing the water. A one-dimensional NMR scanning method was used for quantification of TCE mass distribution within the frozen core. However, the results indicated inconsistency in estimating the total TCE mass within the porous media.
Downhole NMR logging was performed at the former refinery in the western U.S. to detect NAPL and to discriminate NAPL from water in the formation. The results indicated that detection of NMR signals to discriminate NAPL from water is compromised by the noise stemming from the active facilities and/or power lines passing over the site.
A laboratory experiment was performed to evaluate the electrical response of unconsolidated porous media through time (30 days) while NAPL was being depleted. Sand columns (Colorado silica sand) contaminated with methyl tert-butyl ether (MTBE, a light nonaqueous phase liquid (LNAPL)) were studied. A multilevel electrode system was used to measure electrical resistivity of impacted sand by imposing alternative current. The trend of reduction in resistivity through the depth of columns over time followed depletion of LNAPL by volatilization.
Finally, a field experiment was performed at the former refinery in the western U.S. to track natural losses of LNAPL over time. Multilevel systems consisting of water samplers, thermocouples, and electrodes were installed at a clean zone (background zone) and an LNAPL impacted zone. In situ measurements of complex resistivity and temperature were taken and water sampling was performed for each depth (from 3 to 14 feet below the ground surface at one-foot spacing) within almost a year. At both locations, the results indicated decreases in apparent resistivity below the water table over time. This trend was supported by the geochemistry of the pore fluids. Overall, results indicate that application of the electrical resistivity method to track LNAPL depletion at field sites is difficult due to multiple conflicting factors affecting the geoelectrical response of LNAPL-impacted zones over time.
The primary objective of ANSR is the dissemination of technical information on the science behind the characterization and remediation of Light and Dense Non-Aqueous Phase Liquids (NAPLs). Expanding on this goal, the Research Corner has been established to provide research information on advances in NAPL science from academia and similar research institutions. Each issue will provide a brief synopsis of a research topic and link to the thesis/dissertation/report, wherever available.
Related Links
ANSR Archives
Coming Up
Announcements
The ASTM Standard Guide for NAPL Mobility and Migration in Sediments – Evaluation Metrics (E3282-21) is now available!
The ASTM Standard Guide for NAPL Mobility and Migration in Sediments – Screening Process to Categorize Samples for Laboratory NAPL Mobility Testing (E3281-21) is now available!
The ASTM Standard Guide for NAPL Mobility and Migration in Sediment – Sample Collection, Field Screening, and Sample Handling (E3268-20) is now available!
The ASTM Standard Guide for NAPL Mobility and Migration in Sediment – Conceptual Models for Emplacement and Advection (E3248-20) is now available!
API has published the “API LNAPL Transmissivity Workbook Training Video” to assist with baildown test interpretation and identification of frequently encountered problems.
Check them all and join us on the ANSR LinkedIn page for discussion or to share your own tips and tricks!
Upcoming ITRC Training
- March 1: Connecting the Science to Managing LNAPL Sites 3-Part Series: Select/Implement
LNAPL Technologies (Part 3) - March 3: Optimizing Injection Strategies and In Situ Remediation Performance
- March 15: Sustainable and Resilient Remediation
- March 17: TPH Risk Evaluation at Petroleum-Contaminated Sites
- April 7: Harmful Cyanobacterial Blooms (HCBs) Strategies for Preventing and Managing
- April 19: Incremental Sampling Methodology (ISM-2) Update – Q&A Panel Discussion
- May 3: Bioavailability of Contaminants in Soil: Considerations for Human Health Risk Assessment
- May 5: Integrated DNAPL Site Characterization
- May 10: Long-term Contaminant Management Using Institutional Controls
- May 17: 1,4-Dioxane: Science, Characterization & Analysis, and Remediation
Upcoming IPEC Training
- Feb 24: In-Situ Destruction of LNAPL and DNAPL Utilizing Successful Clay Injectability Application
- Feb 24: Surfactant Enhanced Remediation of NAPL, Globule, and Sorbed Phase Contamination Within Soil and Groundwater Regimes
- March 10: Oil Spill Modeling in Rivers: Case Studies
- March 10: Using High Resolution Tools and 3D Modeling Technology to Assess Subsurface Environmental Impacts
- March 24: Molecular Biological Tools: Actionable Data for Petroleum Hydrocarbon Remediation
- March 24: Overland Flow Models and 3DEP Data
- April 7: Environmental Compliance Assurance
- April 7: Produced/Flow-Back Water Recycling/Storage/Evaporation Ponds