Lisa Reyenga, PE, Board Chair, GEI Consultants, Inc.
J. Michael Hawthorne, PG, GEI Consultants, Inc.
Andrew J. Kirkman, PE, BP Corporation North America
Robert Frank, RG, Jacobs
Paul Cho, PG, CA Regional Water Quality Control Board-LA
Randy St. Germain, Dakota Technologies, Inc.
Dr. Terrence Johnson, USEPA
Brent Stafford, Shell Oil Co.
Douglas Blue, Ph.D., Imperial Oil Environmental & Property Solutions (Retired)
Natasha Sihota, Ph.D., Chevron
Kyle Waldron, Marathon Petroleum
Danny D. Reible, Professor at Texas Tech University
Reeti Doshi, National Grid
Mahsa, Shayan, Ph.D., PE, AECOM Technical Services
Chis Marks, Ph.D., Arizona Department of Environmental Quality
Kammy Sra, Ph.D., Chevron
David Edgerton, USEPA
Frequently Asked Questions
DISCLAIMER: This article was prepared by the author(s) in their personal capacity. The opinions expressed in this article are the author’s own and do not necessarily reflect the views of Applied NAPL Science Review (ANSR) or of the ANSR Review Board members.
On January 23, 2023, the EPA released a clarification on the Federal Underground Storage Tank (UST) Regulation on Free Product Removal, 40 CFR 280.64. The clarification provides information on the EPA’s intent for liability owners to remove “free product” to the “maximum extent practicable”. While the clarification directly applies to Federal UST remediation sites, it has implications across the industry. The specific criteria for what free product removal to the maximum extent practicable means in practice was entrusted to the implementing agencies. The term maximum extent practicable has also subsequently been adopted in many state and federal regulations applying to a wide range of hydrocarbon release sites. Therefore, this clarification has the potential to change how this requirement is interpreted across the industry.
When there is a release of petroleum hydrocarbons (e.g., gasoline, diesel), it may impact the soil, soil gas, and/or groundwater underlying the release location. The overall Federal UST regulation details all the requirements to address the release. One aspect of the clarification is to identify that the industry understanding of free product has incorporated new science and become more nuanced. Free product is now characterized as residual, mobile, or migrating LNAPL, consistent with industry best practice documents from the Interstate Technology Regulatory Council, the Cooperative Research Centre for Contamination Assessment and Remediation of the Environment, Contaminated Land: Applications in Real Environments, and others. Each category is further defined in the clarification as:
- Residual – LNAPL that is bound in the soil and will not move into monitoring wells or smear with a rising or falling water table.
- Mobile – LNAPL that exists above residual saturation levels such that it can accumulate in monitoring wells constructed within its footprint or smear vertically with a rising or falling water table but will not migrate or spread from its current footprint (i.e., move into monitoring wells beyond its current footprint).
- Migrating – A LNAPL body that is expanding laterally into areas previously not impacted by LNAPL.
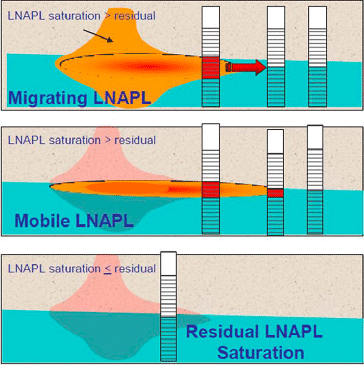
- The federal regulation was written to require owners to remove free product during the early phases of a response to a confirmed release. EPA’s intention was to mitigate the risk of free product spreading to uncontaminated areas of a site.
- UST Sites may have “migrating LNAPL” as well as “mobile LNAPL” and “residual LNAPL.” Mobile and residual LNAPL are both distinct from migrating LNAPL in that they do not spread laterally and do not increase the LNAPL footprint. Abatement of mobile and residual LNAPL is not necessary to meet the requirement of 40 CFR 280.64 to prevent the migration of “free product” into previously uncontaminated soil or groundwater.
Many implementing agencies currently interpret removal to the extent practicable to mean criteria such as removal of any measurable LNAPL or removal to a maximum thickness such as 1/8”, while others may recognize criteria such as diminishing returns (asymptotic recovery) or an LNAPL transmissivity threshold. The intent of these requirements is assumed to be to ensure that the liability owner for the release fulfills their duty to address the release and remediate the impacts. However, in practice, achieving these criteria can have unintended consequences.
A common scenario at hydrocarbon release sites is:
- an older release where the source is no longer active;
- the LNAPL is not migrating and has been stable for many years;
- the LNAPL is weathered and is not contributing to dissolved or vapor phase contaminants that cause a risk to human health or ecological receptors; and
- despite remediation, LNAPL is still present at the site and does not meet the implementing agency’s definition of removal to the maximum extent practicable.
In this scenario, the liability owner may still be required to implement engineered remedial activities. These activities consume resources (e.g., electricity, fuel, or water), produce wastes (e.g., contaminated material for disposal, air emissions, greenhouse gases), yet frequently do not meaningfully improve the environment nor reduce any actual risk. Sites can be stuck in this loop for decades where site closure is not possible under the regulations, but no practical options are available to achieve removal to the maximum extent practicable.
This clarification from the EPA has the potential to change the industry and allow for a holistic, sustainable perspective to be applied more broadly to LNAPL remediation sites. The base requirement of remediation is to abate migration and prevent further spread of contamination. After that, requirements for further remediation can be defined based on mitigating risk to human health and the environment and identifying a sustainable remedial approach. Ultimately, this strategy can guide practitioners towards the least environmental harm resulting from LNAPL releases.
A Word of Caution
References
Interstate Technology and Regulatory Council (2018). Light Non-Aqueous Phase Liquid Site Management: LCSM Evolution, Decision Process, and Remedial technologies. March 2018.
Research Corner

Masters Thesis
University of Waterloo
Abstract
Related Links
ANSR Archives
Coming Up
Announcements
Upcoming ITRC Training
- Nov 7: Recent Advances in PFAS Characterization Technologies
- Nov 7: Microplastics
- Nov 8: Tools for PFAS Site Characterization: Session III – Standards, Passive Sampling, and Modeling of PFAS
- Nov 9: 1,4-Dioxane: Science, Characterization & Analysis, and Remediation
- Nov 14: Conducting Climate Vulnerability Assessments at Superfund Sites: Lessons Learned
- Dec 5: Optimizing Injection Strategies and In situ Remediation Performance
Upcoming IPEC Training
- Nov 9: Waters of the US: What is NEW After the Sackett Case and How Section 106 of the National Historic Preservation Act Relates to Natural Gas Pipeline Permitting
- Dec 5-7: Petroleum Life Cycle: From Rocks to Drill Bits