J. Michael Hawthorne, PG, Board Chairman, GEI Consultants, Inc.
Andrew J. Kirkman, PE, BP Corporation North America
Robert Frank, RG, Jacobs
Paul Cho, PG, CA Regional Water Quality Control Board-LA
Randy St. Germain, Dakota Technologies, Inc.
Dr. Terrence Johnson, USEPA
Brent Stafford, Shell Oil Co.
Douglas Blue, Ph.D., Imperial Oil Environmental & Property Solutions (Retired)
Natasha Sihota, Ph.D., Chevron
Kyle Waldron, Marathon Petroleum
Danny D. Reible, Professor at Texas Tech University
Reeti Doshi, National Grid
Frequently Asked Questions
DISCLAIMER: This article was prepared by the author(s) in their personal capacity. The opinions expressed in this article are the author’s own and do not necessarily reflect the views of Applied NAPL Science Review (ANSR) or of the ANSR Review Board members.
Introduction
Gas ebullition is gas bubble formation and growth in sediment, followed by sediment fracture and the subsequent upward migration of gas bubbles through sediment to the surface water column. Migration of gas bubbles through nonaqueous phase liquid (NAPL) or other organic contaminants in sediments may result in the transport of NAPL/contaminants from sediments to surface water (i.e., ebullition-facilitated transport [EFT]). This can be an important transport mechanism for contaminated sediment sites, moving isolated contaminants into the biologically active zone (Viana and Rockne 2021, 2022 ; Fendinger et al. 1992; Viana et al. 2012, 2018; Yuan et al. 2009). Also, at sites where sediment capping is planned as part of the remedy, ebullition should be evaluated to understand potential impacts to cap design such as contaminant transport and potential gas buildup (which could potentially destabilize a remedial cap).
Flux chambers can be used to accurately quantify EFT rates of NAPL and other contaminants from sediment to the overlying water column. Quantifying the rate of gas production and NAPL/contaminant flux over a representative range of conditions allows for the calculation of annual EFT chemical loads to surface water. These annual loads can then be compared to other contaminant transport mechanisms that may be present at a site and used in remedial design evaluations. ASTM E3300 (ASTM 2021) provides further information on calculation of annual EFT chemical loads.
Near-bottom measurements (i.e., using flux chambers) have proven to better represent gas and contaminant flux than surface-based measurements (Rockne et al. 2011; Viana et al. 2012; Maeck et al. 2014; Zhu et al. 2015). While other methods can be used to measure EFT, this article focuses on the use of near-bottom flux chambers.
Flux Chamber Components
An example flux chamber configuration is illustrated in Figure 1.
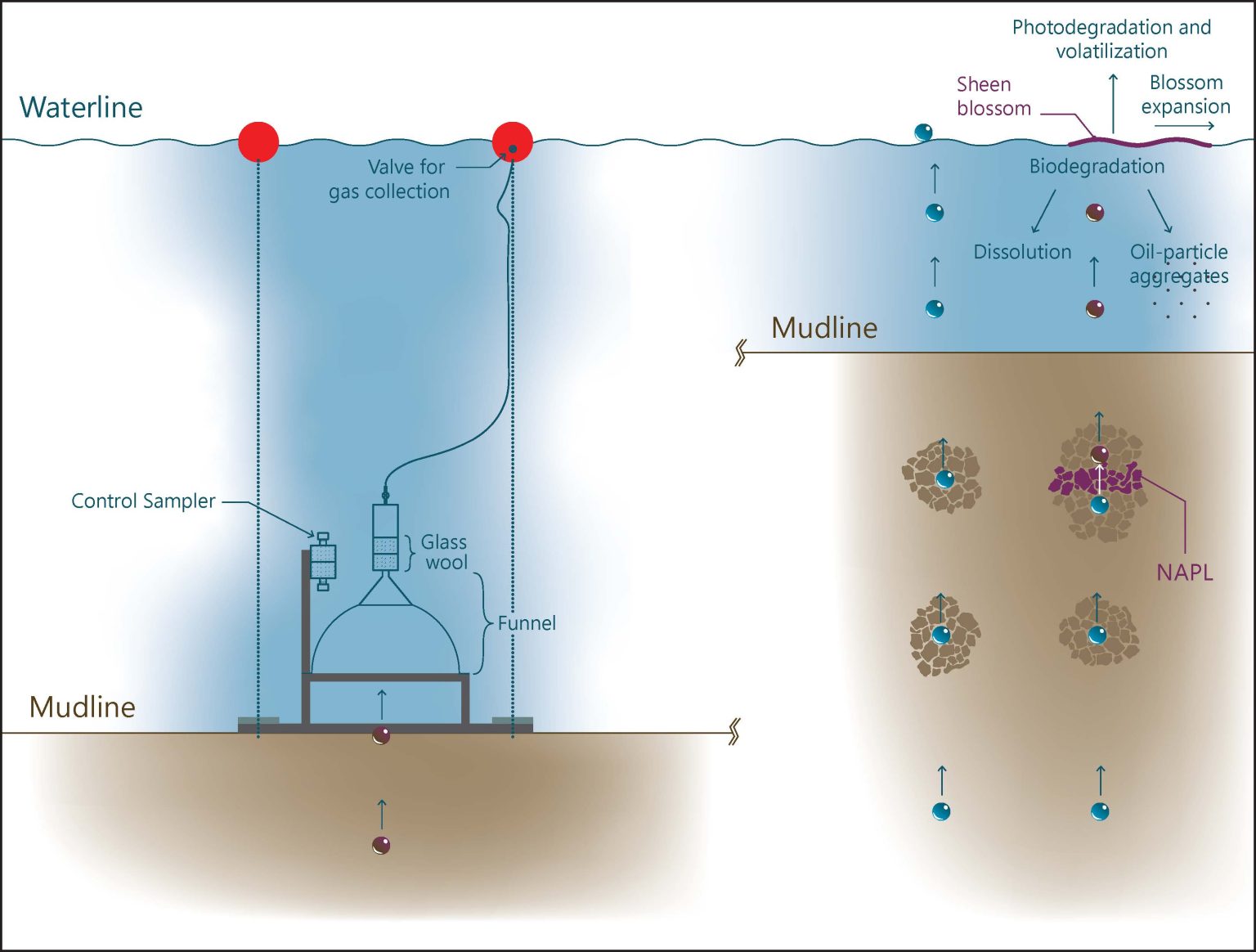
Flux Chamber Field Procedures
Flux chamber locations and deployment timing should be selected to capture a range of gas ebullition conditions within a site. ASTM E3300 (ASTM 2021) provides further information on determining the location of sampling devices, when these devices should be deployed, and the duration of the deployment.
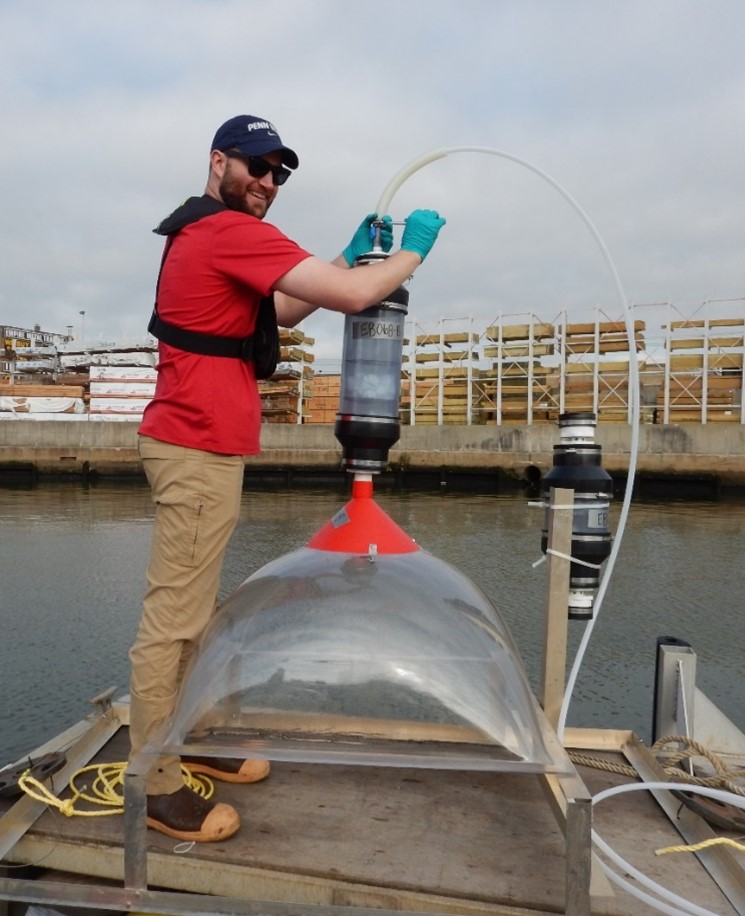
Deployment
Flux chambers should be deployed in a manner that limits sediment disturbance and ensures trapped gas is vented from the system. This objective can be achieved through venting the chamber in the water, slowly lowering it to the mudline, and anchoring it with weights lowered down to the frame. Use of divers is discouraged to avoid potential sediment disturbance. Depending on water depth and clarity, an underwater camera should be used to confirm the submerged flux chamber is properly seated on the sediment surface (i.e., in an upright position).
Maintenance
Flux chambers should be inspected (with the underwater camera if necessary) on a daily basis to ensure correct position (i.e., chambers are not tipped over) and to collect or vent gas. Gas can be collected to measure gas volume or analyze gas composition, or it may be vented. This periodic release of the gas is important to ensure that too much gas does not accumulate in the tubing and back up into the glass wool chamber. Additionally, the temperature and atmospheric pressure should be measured at the time of gas collection. If a flux chamber is found to be tipped over, retrieve and replace with a new flux chamber.
Retrieval and Sample Processing
Flux chamber retrieval should be conducted in a way that minimizes sediment or chamber disturbance to the extent practicable. Gas should be collected (if required) or vented prior to flux chamber retrieval. Once the flux chamber is retrieved from the water, the glass wool compartment and control sampler should be removed and stored for processing. The inside (bottom) surface of the funnel should be cleaned with wipes (with solvent or without, based on the analytical method to be used), and the wipes should be added to the glass wool for sample extraction. Samples should be sent to a laboratory for extraction and analysis for NAPL mass (gravimetric determination) and organics (e.g., polycyclic aromatic hydrocarbons, n-alkanes and isoprenoids, and polychlorinated biphenyls). The sample results can then be used in the calculation of an annual load as described in ASTM E3300 (ASTM 2021).
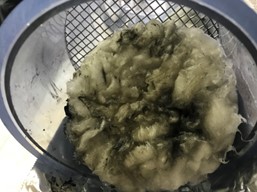
Conclusion
A Word of Caution
These shared best practices are general recommendations, and project-specific considerations may warrant modifications to the methods discussed or consideration of alternate methods.
References
Fendinger, N.J., D.D. Adams, and G.E. Glotfelty, 1992. “The Role of Gas Ebullition in the Transport of Organic Contaminants from Sediments.” Science of Total Environment 112 (2−3):189−201.
Maeck, A., H. Hofmann, and A. Lorke, 2014, “Pumping Methane Out of Aquatic Sediments – Ebullition Forcing Mechanisms in an Impounded River.” Biogeosciences 11:2925–2938.
Rockne, K.J., R. Kaliappan, and G. Bourgon, 2011. Sediment Gas Ebullition Study, Grand Calumet River, Western Branch, Reaches 1 and 2, USACE-Chicago District, 234 pp.
Viana, P., K. Yin, and K. Rockne, 2012. “Field Measurements and Modeling of Ebullition-Facilitated Flux of Heavy Metals and Polycyclic Aromatic Hydrocarbons from Sediments to the Water Column.” Environmental Science and Technology 46(21):12046–12054.
Viana, P., K. Yin, and K. Rockne, 2018. “Comparison of Direct Benthic Flux to Ebullition-Facilitated Flux of Polycyclic Aromatic Hydrocarbons and Heavy Metals Measured in the Field.” Journal of Soils and Sediments 18:1729–1742.
Viana, P.Z. and K.J. Rockne, 2022. “Assessment and Control of Ebullition Facilitated NAPL and Contaminant Transport in Sediment.” Applied NAPL Science Review 10(3). May 2022.
Viana, P.Z. and Rockne, K. J., 2021. “Fundamentals of Ebullition Facilitated NAPL and Contaminant Transport.” Applied NAPL Science Review 9(6). September 2021.
Yuan, Q., K.T. Valsaraj, and D.D. Reible, 2009. “A Model for Contaminant and Sediment Transport via Gas Ebullition Through A Sediment Cap.” Environmental Engineering Science 26(9):1381−1391.
Zhu, T., D. Fu, C.T. Jafvert, and R.P. Singh, 2015. “Modeling of Gas Generation from the River Adjacent to the Manufactured Gas Plant.” RSC Advances 5:9565–9573.
Research Corner

Master of Science
Colorado State University
Abstract
Related Links
ANSR Archives
Coming Up
Announcements
Upcoming ITRC Training
• September 20: Characterization and Remediation in Fractured Rock
• September 22: Remediation Management of Complex Sites
Upcoming IPEC Training
• October 4-6: Petroleum Life Cycle: From Rocks to Drill Bits