J. Michael Hawthorne, PG, Board Chairman, GEI Consultants, Inc.
Andrew J. Kirkman, PE, BP Corporation North America
Robert Frank, RG, Jacobs
Paul Cho, PG, CA Regional Water Quality Control Board-LA
Randy St. Germain, Dakota Technologies, Inc.
Dr. Terrence Johnson, USEPA
Brent Stafford, Shell Oil Co.
Douglas Blue, Ph.D., Imperial Oil Environmental & Property Solutions (Retired)
Natasha Sihota, Ph.D., Chevron
Kyle Waldron, Marathon Petroleum
Danny D. Reible, Professor at Texas Tech University
Reeti Doshi, National Grid
Frequently Asked Questions
DISCLAIMER: This article was prepared by the author(s) in their personal capacity. The opinions expressed in this article are the author’s own and do not necessarily reflect the views of Applied NAPL Science Review (ANSR) or of the ANSR Review Board members.
Karl J. Rockne, Ph.D., PE, BCEE, University of Illinois at Chicago
The migration of gas bubbles through sediment and surface water is called gas ebullition. In many organic-rich sediment environments, microorganisms can produce gas comprised of methane with a typical range from 40 to 90%, and lesser amounts of carbon dioxide and other gaseous end products (Casper 2000, Huttunen et al. 2001, Rockne et al. 2011, and Viana et al. 2012). Gas ebullition requires that the gas production rate must be sufficiently rapid to cause gas bubbles to grow, fracture the sediment, and subsequently migrate upward (Boudreau 2012, Zamanpour et al. 2020). Gas ebullition can represent an important mass transport mechanism of NAPL and/or other contaminants to the water column (Fendinger et al. 1992, Viana et al. 2012, Viana et al. 2018, Yuan et al. 2009, Zamanpour et al. 2020). Viana and Rockne (2021) summarize the mechanisms of biogenic gas production in sediment, ebullition processes, and NAPL transport via ebullition. They also provide a summary of site-specific conditions and characteristics that affect gas production, gas ebullition, and associated NAPL and contaminant transport.
Evaluation of the presence of gas ebullition and its potential to serve as a mechanism for NAPL transport is a critical step in contaminated site characterization, conceptual site model development, assessment of remedial alternatives, and sediment remediation design. This paper summarizes methods to assess gas ebullition, ebullition facilitated NAPL and contaminant transport, and discuss the implications of these processes for sediment remediation.
Screening Tools
Several investigative approaches and modeling tools have been developed to screen sites for gas ebullition potential, as well as to quantify or estimate gas ebullition flux, ebullition facilitated NAPL and contaminant flux, and total flux for remedial design purposes. These approaches include:
- Models. Several empirical and mechanistic models for gas ebullition have been reported in the literature (e.g., Fendinger et al. 1992, Mongollon et al. 2009, Viana et al. 2012, Winterwerp and VanKestern 2004, Yuan et al. 2009, and Zamanpour and Rockne 2018). These can be used to estimate gas ebullition rate and associated transport of contaminants to the water column for assessing the potential for gas ebullition facilitated NAPL and contaminant transport at a site. Model inputs include:
- Sediment properties including chemical concentrations, porosity, density, total organic carbon content, organic matter degradability, sheer strength, and compression strength,
- Physical parameters including temperature and water depth, and
- Biological properties affecting gas production such as degradation rate and porewater chemical concentrations.
- Ebullition surveys. Field assessments may include:
- Evaluation of potential occurrence of gas ebullition by collecting evidence of ebullition-facilitated transport of NAPL to the water column including field observation of sediment particle resuspension and/or visual observations of sheen blossoms when the gas bubble breaks at the water surface, and
- Counting gas bubble frequency per unit area to estimate gas ebullition rates and assess frequency and spatial extent of ebullition events and/or underwater observation using cameras or divers.
- Bench-scale tests. These may be used as initial screening tool(s) or to provide data for modeling to evaluate the potential for gas production. The main objective of this initial screening is to evaluate if gas production may occur with sufficient magnitude and prevalence to represent an important contaminant transport mechanism and/or impact remedy performance.
Quantitative Measurements
If there is indication that gas ebullition is an important transport mechanism, measurement of the rate and quantity of in-situ volumetric gas release and associated NAPL transport may be conducted to quantify the contaminant mass flux from the sediment to the water column. Gas flux measurements should be collected simultaneously with associated NAPL and contaminant flux measurements at each sample location, whenever possible. ASTM E3300-21 provides a list of measurement methods and their advantages and limitations. Figure 1 below provides a sketch of a gas ebullition flux chamber used by Viana et al. (2012), while Figure 2 shows a photograph of a tent sampler used by Zhu et al. (2015).
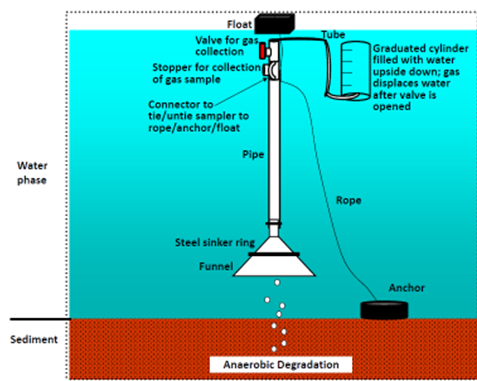
Above Sediment/Surface Water Interface
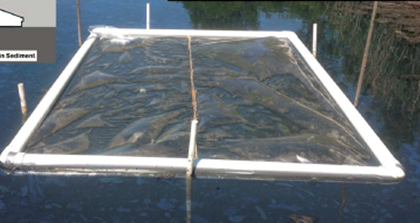
at Air/Surface Water Interface
- Minimizing the distance between the sampler and the sediment surface,
- Careful sampler placement and anchoring to minimize sediment disturbance,
- Proper preservation and rapid analysis of gas composition samples, and
- Collection and analysis of appropriate control samples.
Table 1 provides a summary of several reports of gas ebullition fluxes reported in the literature, while Table 2 presents ebullition facilitated contaminant flux data reported in the literature. Two observations are made from these data. First, reported gas fluxes range by a factor of 100 or more. Second, very few reports in the literature have quantified the flux of contaminants facilitated by ebullition.
Location | Number of Samplers / Measurements | Gas Flux Rates (L m-2 d-1) | Sampler Size | Measurement Timeframe | Source |
---|---|---|---|---|---|
Chicago River, IL | 14 locations/168 (14 locations x minimum 3 days x 4 seasons) | 0.05 to 8.6 | 0.05 m2 | Minimum of 3 days per sampling event | Viana et al. (2012) |
Grand Calumet River, IN | 13 locations/Pre-capping investigation | 0.1 to 6.7 | 0.03 or 0.05 m2 | Minimum of 3 days per sampling event | Rockne et al. (2011) |
Grand Calumet River, IN | 12 locations/14 samplers after placement of a reactive cap | 0 to 1.8 | 0.03 or 0.05 m2 | Minimum of 3 days per sampling event | Rockne and Kaliappan (2013) |
Constructed wetlands treating wastewater | 4 locations/ 2 samplers per location | 0.07 to 0.67 (methane) | 0.25 m2 | Summer (automated flux chamber) | Tanner et al. (1997) |
Constructed wetlands, Norway | 3 ponds/3 samplers per pond | 0 to 3.4 (methane) | 0.25 m2 | Autumn, winter, and summer | Sovik and Klove (2007) |
Lake Kinneret, Israel | Hydroacoustic technology (dual-beam 120 kHz echosounder) quantified gas bubble abundance over time, 14 acoustic transects | 0.23 | NA | Data collected during summer | Ostrovsky et al. (2008) |
Location | Number of Samplers / Measurements | Contaminant Flux Rates (mg m-2 d-1) | Sampler Size | Measurement Timeframe | Source |
---|---|---|---|---|---|
Chicago River, IL | 168 discrete sample measurements at 14 different locations | 50 ± 13 (Fe) 2.6 ± 0.71 (Zn) 1.5 ± 0.28 (Pb) 0.19 ± 0.06 (Cr) 0 to 240 (total PAHs) | 0.05 m2 | Minimum of 3 days per sampling event | Viana et al. (2012) |
Grand Calumet River, IN | 13 locations/Pre-capping investigation | Sum of 7 metals: 10 to 50 Sum of 14 PAHs: 0.15 to 0.92 | 0.03 or 0.05 m2 | Minimum of 6 days per sampling event | Rockne et al. (2011) |
Grand Calumet River, IN | 12 locations/14 samplers post placement of a reactive cap | Sum of 7 metals: <5 Sum of 14 PAHs: typically, below detection limit | 0.03 or 0.05 m2 | Minimum of 6 days per sampling event | Rockne and Kaliappan (2013) |
Impacts for Sediment Remediation
Measurement or modeling of the spatial occurrence and rate of gas ebullition is important for conceptual model development and assessment of remedial alternatives where impacted sediments remain in situ. More recently, researchers are investigating the utility of using machine language approaches to predict gas ebullition from more readily obtainable sensor data (Mansouri and Rockne, 2022).
The use of these models as predictive tools can be challenging because of several factors including:
- Availability of suitable data,
- Spatial and temporal variability of ebullition-facilitated NAPL/contaminant transport, and
- Uncertainty associated with the predictions using different models.
Gas ebullition and associated NAPL and contaminant transport facilitated by gas ebullition can affect the performance of a sediment remedy where NAPL and contaminants are left in place. For example, NAPL/contaminant transport facilitated by gas ebullition from underlying sediments can result in consumption of sorption capacity in a sediment treated using sorptive amendments, or in a sediment cap containing sorptive amendments. In addition, gas ebullition may fracture and/or burst low porosity and low permeability caps, resulting in the mobilization of NAPL and contaminants in sediments underlying the remedy layer, and opening of preferential pathways to the surface water for contaminant release. Therefore, remedial alternatives such as capping, amended residuals cover layer, and in-situ amendment should be designed taking into consideration the potential for gas ebullition and associated NAPL and contaminant transport.
While sediment capping or an amended residuals cover layer is likely to reduce ebullition-facilitated NAPL transport by preventing resuspension and by enhancing sediment strength to prevent fracture, ebullition-facilitated NAPL and/or contaminant transport from buried biodegradable organic matter may still result from:
- Hydrophobic contaminants in sediment porewater that partition into the gas phase,
- NAPL that may accumulate on the surface of gas bubbles and be transported laterally or upward through fracture networks,
- Sediment disturbance during remedy placement that releases entrained gas, and/or
- Sediment, residuals cover, or capping erosion which could release entrained gas.
Prevention of the microbial processing of organic matter in sediments without removing the biodegradable organic matter that drives the process is challenging, if not impossible. However, if the primary source of biodegradable organic matter is deposition to the sediment surface, ebullition may be reduced over time as this organic matter is degraded. In such cases, ebullition facilitated contaminant flux to the water column may decrease if source control can prevent contamination of the ebullition-active zone in the sediment.
Based on the current state of knowledge, we propose that in-situ remedial options for ebullition active sites should consider the following primary issues for addressing the problem of gas ebullition facilitated contaminant flux:
- Control of organic matter biodegradation to decrease the biogenic gas production rate,
- Manipulation of sediment geophysical conditions to strengthen the sediment and prevent fracturing, and/or
- Mitigation of ebullition-facilitated transport of NAPL and other contaminants to surface water through entrapment, treatment, or a combination of the two processes prior to release of gas bubbles to surface water through an in-situ treatment layer.
Appropriate design of in-situ treatment remedies accounting for gas ebullition in active gas ebullition sites is key for successful sediment remediation.
A Word of Caution
References
Boudreau, B.P., 2012. The Physics of Bubbles in Surficial, Soft, Cohesive Sediments. Marine and Petroleum Geology 38:1–18.
Casper, P., S.C. Maberly, G.H. Hall, and B.J. Finlay. 2000. Fluxes of CH4 and CO2 from a Small Productive Lake to the Atmosphere. Biogeochemistry 49(1): 1.
Fendinger, N. J., Adams, D. D., Glotfelty, D. E., 1992. The Role of Gas Ebullition in the Transport of Organic Contaminants from Sediments. Science of Total Environment 112 (2−3): 189−201.
Huttunen, J.T., K.M. Lappalainen, E. Saarijärvi, T. Väisänen, and P.J. Martikainen, 2001. A Novel Sediment Gas Sampler and A Subsurface Gas Collector Used For Measurement Of The Ebullition of Methane and Carbon Dioxide from A Eutrophied Lake. Science of the Total Environment 266(1-3): 153.
Kaliappan, R. and K. Rockne, 2015. Estimating Post-Capping GW-SW Exchange At The Grand Calumet River Using Streambed Temperature Profiles. In: Eighth International Conference on the Remediation and Management of Contaminated Sediments, January 13-15, New Orleans, Louisiana, 9 pp.
Mansouri, M. and K. J. Rockne, 2022. Machine Learning Approaches To Predict Gas Ebullition From Sediments. In: Emerging Contaminants in the Environment Conference. The Illinois Sustainable Technology Center and Illinois-Indiana Sea Grant, April 27–28, Urbana, IL.
Mogollon, J. M., L’Heureux, I., Dale, A. W., Regnier, P, 2009. Methane Gas-Phase Dynamics In Marine Sediments: A model study. American Journal of Science 309 (3): 189−220.
Ostrovsky, I., D.F. McGinnis, L. Lapidus, W. Eckert, 2008. Quantifying Gas Ebullition With Echosounder: The Role Of Methane Transport By Bubbles In A Medium-Sized Lake. Limnology Oceanography Methods 2008, 6, 105-118.
Rockne, K. J., R. Kaliappan, G. Bourgon, 2011. Sediment Gas Ebullition Study, Grand Calumet River, Western Branch, Reaches 1 and 2, USACE-Chicago District, 234 pp.
Rockne, K. J., and R. Kaliappan, 2013. Post-Capping Sediment Gas Ebullition Study, Grand Calumet River, Western Branch, Reaches 1 and 2, USACE-Chicago District, 157 pp.
Sovik, A.K. and B. Klove, 2007. Emission of N2O and CH4 from a Constructed Wetland in Southeastern Norway. Science of the Total Environment 380:28-37.
Tanner, C.C., D.D. Adams, and M.T. Downes, 1997. Methane Emissions from Constructed Wetlands Treating Agricultural Wastewaters. Journal of Environmental Quality 26(4): 1056 – 1062.
Viana, P., K. Yin, and K. Rockne, 2012. Field Measurements and Modeling of Ebullition-Facilitated Flux of Heavy Metals and Polycyclic Aromatic Hydrocarbons from Sediments to the Water Column. Environmental Science and Technology 46(21): 12046-12054.
Viana, P., K. Yin, and K. Rockne, 2018. Comparison of Direct Benthic Flux to Ebullition-Facilitated Flux of Polycyclic Aromatic Hydrocarbons and Heavy Metals Measured in the Field. Journal of Soils and Sediments 18: 1729-1742.
Viana, P. and K. Rockne, 2021. Fundamentals of Ebullition Facilitated NAPL and Contaminant Transport. Applied NAPL Science Review 9(6), September.
Winterwerp, J. C., Van Kesteren, W. G. M, 2004. Introduction to the Physics Of Cohesive Sediment In The Marine Environment. Elsevier.
Yuan, Q., K. T. Valsaraj, and D. D. Reible, 2009. A Model for Contaminant and Sediment Transport via Gas Ebullition Through A Sediment Cap. Environmental Engineering Science 26 (9): 1381−1391.
Zamanpour, M. K. and K. J. Rockne, 2018. A Mechanistic Model for Gas Ebullition in the Presence of NAPLs in Sediments. In: World Environmental and Water Resources Congress, June 3–7, Minneapolis, Minnesota.
Zamanpour, M. K., R. S. Kaliappan, and K. J. Rockne, 2020. Gas Ebullition from Petroleum Hydrocarbons in Aquatic Sediments; A Review. Journal of Environmental Management 271: 110997.
Zhu, T., D. Fu, C. T. Jafvert, and R. P. Singh, 2015. Modeling of Gas Generation from the River Adjacent to the Manufactured Gas Plant. RSC Advances 5: 9565.
Research Corner

Master of Science
Colorado State University
Abstract
Related Links
ANSR Archives
Coming Up
Announcements
Upcoming ITRC Training
- June 2: Vapor Intrusion Mitigation Session 1: Conceptual Site Model for Vapor Intrusion Mitigation, Public Outreach, Rapid Response, Remediation & Long-term Contaminant Management Using Institutional Controls
- June 14: Vapor Intrusion Mitigation Session 2: Active Mitigation, Passive Mitigation, Installation/ OM&M/Exit Strategy
Upcoming IPEC Training
- June 2: Mixed Chlorinated & Hydrocarbon In-Situ Destruction in a Single Scope of Work Solar AS/SVE Remediation Systems
- June 16: Surfactant Enhanced Extraction of Semi-Volatile and Volatile Contaminants Within Soil and Groundwater Regimes