J. Michael Hawthorne, PG, Board Chairman, GEI Consultants, Inc.
Andrew J. Kirkman, PE, BP Corporation North America
Robert Frank, RG, Jacobs
Paul Cho, PG, CA Regional Water Quality Control Board-LA
Randy St. Germain, Dakota Technologies, Inc.
Dr. Terrence Johnson, USEPA
Brent Stafford, Shell Oil Co.
Douglas Blue, Ph.D., Imperial Oil Environmental & Property Solutions (Retired)
Natasha Sihota, Ph.D., Chevron
Kyle Waldron, Marathon Petroleum
Danny D. Reible, Professor at Texas Tech University
Reeti Doshi, National Grid
Mahsa, Shayan, Ph.D., PE, AECOM Technical Services
Frequently Asked Questions
DISCLAIMER: This article was prepared by the author(s) in their personal capacity. The opinions expressed in this article are the author’s own and do not necessarily reflect the views of Applied NAPL Science Review (ANSR) or of the ANSR Review Board members.
Introduction
Aqueous film-forming foam (AFFF) contains high concentrations (grams per liter) of per- and polyfluoroalkyl substances (PFAS) and was historically used to combat fires where the fuel source was non-aqueous phase liquids (NAPLs) including light non-aqueous phase liquids (LNAPLs) such as diesel, gasoline, or waste oil and/or dense non-aqueous phase liquids (DNAPLs) such as trichloroethene (TCE) (Figure 1). These events occurred at fire-training areas, fuel spill locations, in hangars or on runways, and at bulk fuel storage areas. Conceptually, after being sprayed on the fire, AFFF foam eventually collapsed and AFFF liquid infiltrated the subsurface. PFAS dissolved in water also infiltrated the subsurface. Although several studies showed preferential association of PFAS to NAPL and at the air-water interface (AWI), evidence from one study indicated PFAS can form an immobile LNAPL/PFAS microemulsion (LPME) with milligrams per liter (mg/L) concentrations of PFAS (Figure 2) at the NAPL-water interface (NWI). PFAS retained in NAPL, at the AWI, or as LPME may represent an ongoing source of PFAS to groundwater and limit disposal options for recovered LNAPL, which was previously treated through traditional means that are likely ineffective for PFAS treatment. PFAS persistence in the subsurface and potential treatment difficulty can increase remedial durations and associated costs. Therefore, understanding the presence and behavior of PFAS at sites with NAPL or where LPME may have formed is necessary for site characterization, remediation, and revitalization.
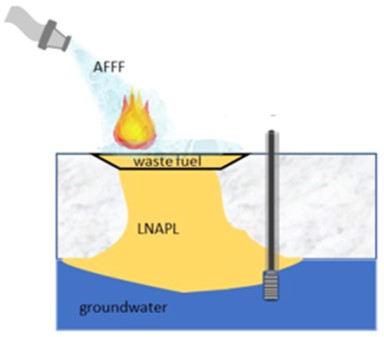
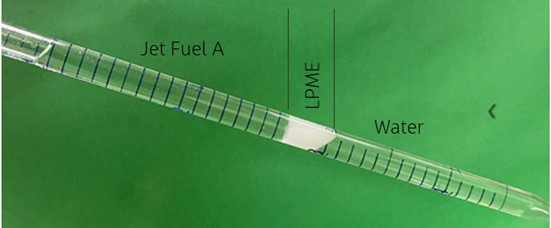
where the interface between the upper and middle phases remains when the pipette is tilted.
Laboratory Studies
Four laboratory studies reviewed indicate the potential for PFAS retention to NAPL and at the AWI and NWI. A brief summary of each study and associated results relevant to this article are summarized in the following table. More recent studies confirm these earlier findings (Brusseau and Van Glubt [2021], Brusseau [2021], Silva et al. [2019]).
Reference | Study Brief* | Relevant Conclusions(s)* |
---|---|---|
Guelfo and Higgins (2013) | Batch sorption experiments of transport potential of perfluoroalkyl acids (PFAAs) in different soils, and soil with TCE. | TCE acted as a PFAS sorbent in soil with low organic carbon content. |
McKenzie et al. (2016) | 1-D column experiments with vertical up-flow through loamy sand with TCE to assess PFAA transport. | TCE provided additional adsorption capacity/increased PFAA retardation. |
PFAA were transferred to the NAPL phase. | +1 if NAPL saturation would be reduced to immobile saturation before reaching receptor (for example, surface sediment) -1 if NAPL saturation would not be reduced to immobile saturation before reaching receptor | If the NAPL is depleted to immobile saturation before encountering a receptor, it will not be able to continue migrating toward that receptor. If the calculation shows otherwise, it may be possible for the NAPL to migrate far enough to reach the receptor. |
Brusseau (2018) | Used data collected from literature model and assess the importance and magnitude of perfluorooctanesulfonic acid (PFOS) and perfluorooctanoic acid (PFOA) retention processes. | PFAS retardation includes multiple processes. Adsorption at the AWI in sandy soil contributed to 50 percent of the retention. |
The LPME was observed to be thermodynamically stable with transport properties very different from those of the LNAPL or AFFF alone (Dwarakanath et al., 2000). Kostarelos, et al. (2021) note that the LPME that formed had a viscosity 110 times that of water and significantly higher than those of Jet Fuel A and AFFF-solution as shown on Figure 3.
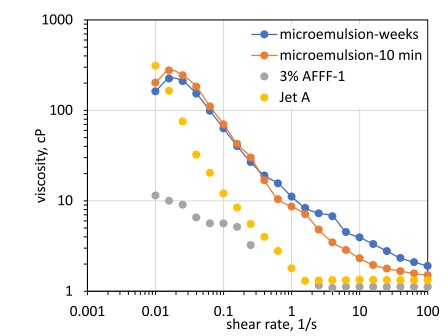
(chart from Kostarelos et al. [2021])
Relevance
Because AFFF was routinely used to douse NAPL fires, these contaminants have interacted in the subsurface at many sites. Based on the laboratory evidence, PFAS retention in the subsurface at the AWI, NWI, within residual NAPL, or as LPME may be a relatively common occurrence. To date, remediation at these sites targeted NAPL recovery or treatment without consideration of PFAS. In particular, LNAPL skimming or enhanced oil recovery technologies were not designed to mobilize and extract LPME with physical properties different from the LNAPL alone. In addition, at sites where LNAPL is extracted and treated ex-situ, the presence of PFAS in the LNAPL likely affects treatability and disposal options. Therefore, site characterization and remedial designs need to understand and account for PFAS behavior in the subsurface at sites with co-located releases of NAPL.
Summary
Laboratory evidence suggests that PFAS may be retained in the subsurface at sites where NAPL was also released likely resulting in a persistent source. In addition, the formation of LPME may complicate existing remediation strategies at LNAPL sites currently using methods such as extraction and offsite disposal. With the recent development of an analytical method at Oregon State University (Jennifer Field, 2022, Personal Communication) to quantify PFAS in NAPL, these sites may need to be re-evaluated for the presence of residual PFAS mass in NAPL that may be acting as a long-term source to groundwater. Further study is needed to identify the conditions that affect PFAS retention, how common those conditions are, better understand their retention mechanisms, and evaluate technologies to treat the PFAS-impacted media. In addition, at sites where NAPL has been extracted and later found to contain PFAS, ex situ technologies to separate PFAS from NAPL require study.
A Word of Caution
References
Brusseau, M. L., Examining the robustness and concentration dependency of PFAS air-water and NAPL-water interfacial adsorption coefficients. Water Res. 2021, 190.
Brusseau, M. L. and Van Glubt, S., The influence of molecular structure on PFAS adsorption at air-water interfaces in electrolyte solutions. Chemosphere, 2021, 281.
Dwarakanath, V.; Pope, G. A. Surfactant phase behavior with field degreasing solvent. Environ. Sci. Technol. 2000, 34 (22), 4842−4848.
Guelfo, J. L. and Higgins, C. P., Subsurface transport potential of perfluoroalkyl acids at aqueous film-forming foam (AFFF)-impacted sites. Environ. Sci. Technol. 2013, 47, (9), 4164-4171.
Kostarelos, K.; Sharma, P.; Christie, E.; Wanzek, T.; Field, J., Viscous microemulsions of aqueous film-forming foam (AFFF) and Jet Fuel A inhibit infiltration and subsurface transport. Environ. Sci. Technol. Lett. 2021, 8, (2), 142-147.
McKenzie, E. R.; Siegrist, R. L.; McCray, J. E.; Higgins, C. P., The influence of a non-aqueous phase liquid (NAPL) and chemical oxidant application on perfluoroalkyl acid (PFAA) fate and transport. Water Res. 2016, 92, 199-207.
Silva, J. A. K.; Martin, W. A.; Johnson, J. L.; McCray, J. E., Evaluating air-water and NAPL- water interfacial adsorption and retention of Perfluorocarboxylic acids within the Vadose zone. J Contam Hydrol. 2019, 223.
Van Glubt, S. and Brusseau, M. L., Contribution of nonaqueous-phase liquids to the retention and transport of per and polyfluoroalkyl substances (PFAS) in porous media. Environ. Sci. Technol. 2021, 55, (6), 3706-k.
Research Corner

Doctor of Philosophy
Oregon State University
Abstract
Related Links
ANSR Archives
Coming Up
Announcements
Concawe (CONservation of Clean Air and Water in Europe) published the LNAPL Toolbox. The LNAPL Toolbox is “a unique collection of useful tools, calculators, data, and resources to help LNAPL scientists and engineers better understand how to manage LNAPL at their sites.”
Upcoming ITRC Training
- September 13: Optimizing Injection Strategies and In Situ
- September 20: Characterization and Remediation in Fractured Rock
- September 22: Remediation Management of Complex Sites
- October 18: 1,4-Dioxane Science, Characterization & Analysis, and Remediation
- November 3: Vapor Intrusion Mitigation Session 1, Conceptual Site Model for Vapor Intrusion Mitigation, Public Outreach, Rapid Response, Remediation & Long-term Contaminant Management Using Institutional Controls
- November 8: Harmful Cyanobacterial Blooms (HCBs) Strategies for Preventing and Managing
- November 15: Vapor Intrusion Mitigation Session 2, Active Mitigation, Passive Mitigation, Installation/ OM&M/Exit Strategy
- November 17: Sustainable and Resilient Remediation
- December 6: Optimizing Injection Strategies and In Situ Remediation Performance
Upcoming IPEC Training
- August 25: Clean Air Update: Impact of Recent Court Cases /Bioremediation 4.0: What Procaryotic Microbes can really accomplish and the roll QSS Plays
- September 8: In-Situ Destruction of LNAPL Utilizing Successful Clay Injectability Application / New Aqueous Based Solutions Promote Waste Minimization and Biodiversity Protection: Case Study
- September 22: Floodplain 101 / Site Assessment for Oil & Gas Facilities and Associated Infrastructure
- October 6: In-Place Treatment of Hydrocarbon Releases Signals a Breakthrough in the Conservation of Top Soil & Vegetation / Oil and Gas ESG Best Practices
- October 20: A New Tool for the Assessment of Natural Source Zone Depletion / Primary Criteria Required to Design Successful UST Remediation Projects
- November 3: Orphan Wells: A Best Practices Approach / Beyond the E in ESG for Oil & Gas
- November 17: Using UV Fluorescence Tools to Investigate Subsurface Contamination at a former MGP / Anaerobic Degradation of PHC through Biostimulation Induced Biofilm Development
- December 1: Collaborative In-Situ Design Criteria and Strategy for Maximum Application Success / Surfactant Enhanced Extraction (SEE) of VOC, Sorbed, and NAPL Phases Exposing Factors which Limit their Remediation