J. Michael Hawthorne, PG, Board Chairman, GEI Consultants, Inc.
Andrew J. Kirkman, PE, BP Corporation North America
Robert Frank, RG, Jacobs
Paul Cho, PG, CA Regional Water Quality Control Board-LA
Randy St. Germain, Dakota Technologies, Inc.
Dr. Terrence Johnson, USEPA
Brent Stafford, Shell Oil Co.
Douglas Blue, Ph.D., Imperial Oil Environmental & Property Solutions (Retired)
Natasha Sihota, Ph.D., Chevron
Kyle Waldron, Marathon Petroleum
Danny D. Reible, Professor at Texas Tech University
Reeti Doshi, National Grid
Mahsa, Shayan, Ph.D., PE, AECOM Technical Services
Frequently Asked Questions
DISCLAIMER: This article was prepared by the author(s) in their personal capacity. The opinions expressed in this article are the author’s own and do not necessarily reflect the views of Applied NAPL Science Review (ANSR) or of the ANSR Review Board members.
Introduction
Sediment has historically been a sink for legacy pollutants contained in industrial discharges to surface water. These pollutants have primarily been polychlorinated biphenyls (PCBs), polynuclear aromatic hydrocarbons (PAHs), metals, organic solvents and non-aqueous phase liquids (NAPL). However, over the past several years perfluoroalkyl and polyfluoroalkyl substances (PFAS) began receiving attention for their presence in soil, groundwater and air and the focus later expanded to sediment.
PFAS have been observed to co-occur with NAPLs such as chlorinated solvents and hydrocarbon fuels (Van Glubt and Brusseau, 2021) in soils and sediment. The presence of NAPL appears to impact the transport and distribution of the PFAS in the environment. As surfactants, PFAS have a tendency to be adsorbed or concentrated on air-water or NAPL-water interfaces (Sima and Jaffé, 2021) but also partitions into bulk NAPL. The relative contributions of bulk solid-phase adsorption versus NAPL−water interfacial adsorption vary, depending on the specific PFAS and on system conditions, including sediment or soil type, NAPL saturation and solution properties. This has been found to be the case for systems with even differing NAPL types, NAPL saturation levels, input concentrations, and pore−water velocities.
To assess the impact of anthropogenic chemicals in natural aquatic systems, there are several key pieces of information that scientists typically use. These include the nature of the specific chemicals present, potential sources and chemical properties. Some of the key chemical properties include partition coefficients and biota-sediment accumulation factor for sediment sites. There are also chemical-specific sampling protocols to follow and developing health advisory levels to target. A current understanding of several of these characteristics is discussed below.
What are PFAS?
PFAS are a group of chemicals that were first developed in the 1930s. They have been used in a wide range of applications because of their many favorable properties. Among these properties are flame suppression, electrical insulation, preventing corrosion and repelling moisture. Two PFAS of most prominent concern in the environment are Perfluorooctanoic Acid (PFOA) and Perfluorooctane Sulfonic Acid (PFOS) which have historically been used in making a wide range of consumer products. These products include carpets, clothing, fabrics for furniture, and packaging for food and cookware that are resistant to water, grease or stains. They are also used in aqueous film forming foam for firefighting at airfields and other locations and in a number of other industrial processes (USEPA 2022).
Sources of PFAS to Sediment
PFAS in sediments primarily comes from contaminated groundwater plumes moving through the sediments and particle-associated PFAS from sources such as stormwater settling and depositing on sediments, direct discharges, leachate from wastewater treatment plant biosolids and landfills, and atmospheric deposition. Of the two most prominent classes of PFAS, PFOS is more associated with solids relative to PFAS. Examples of the transfer of PFAS mass from groundwater to surface water over several years were seen in five tributaries at the Cape Fear River in North Carolina (USA) near a facility that manufactured PFAS (Pétré, 2021), where concentrations of PFAS in surface water were similar to those in groundwater. In waterways, these chemicals disperse in many directions including vertically in response to surface water velocity changes. In general, the longer chain compounds tend to partition preferentially to the sediments. The air-borne transport contribution is limited as an air quality model predicted that 5% of emitted PFAS mass from a North Carolina (USA) fluoropolymer factory were deposited within an area up to approximately 150 kilometers away (D’Ambro et al., 2021).
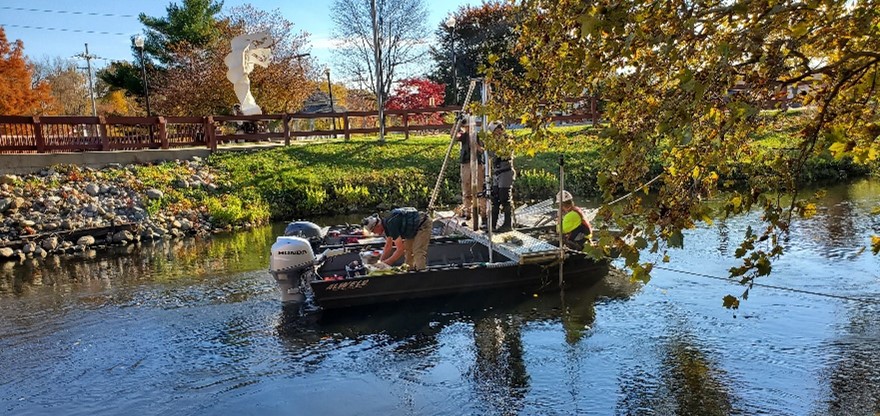
Sampling and Analysis
An example of sediment sampling involving the collection of cores is presented in Figure 1. Due to its ubiquitous presence, specific sampling protocols are required for PFAS-contaminated sediment to avoid cross-contamination. Therefore, PFAS-specific sampling guidance have been developed by various entities. For example, the State of Michigan (USA) has developed a twelve-page Sediment PFAS Sampling guidance that discusses the processes, decontamination procedures, and acceptable materials for samping sediment for PFAS (MDEQ, 2018). The guidance also identifies types of materials to avoid and others that need screening. The document notes that USEPA SW846 Method 537 is used to analyze for PFAS but only applies to drinking water samples and recommends ASTM methods for soil and sediment matrices. The two available ASTM methods are D7968: Standard Test Method for Determination of Perfluorinated Compounds in Soil by Liquid Chromatography Tandem Mass Spectrometry and D7979: Standard Test Method for Determination of Perfluorinated Compounds in Water, Sludge, Influent, Effluent and Wastewater by Liquid Chromatography Tandem Mass Spectrometry.
Data Evaluation
Several of the processes that are typically evaluated at sediment sites are not fully understood but ongoing research continue to contribute new information. For example, sorption coefficients Kd for three PFAS including PFOA, perfluorohexane sulfonic acid (PFHxS) and PFOS in coastal sediments from nineteen locations were determined by Oliver et al. (2020). The median Kd values all varied linearly with the sediment organic carbon content which is an important observation. Consideration is also being given to developing guidelines for protecting benthic invertebrates from PFOS in sediment but there is a paucity of toxicity data available to go beyond preliminary values. More research projects are needed to provide a better understanding of biomagnification, whether total organic carbon (TOC) can be used consistently to normalize PFOS concentrations, PFAS toxicity and bioaccumulation so as to better predict the effects of PFAS mixtures. Basic trophic web models and equilibrium partitioning have been used to develop initial quality guidelines to apply to sediment for human health and wildlife protection in Switzerland (Casado-Martinez et al., 2021). However, the biomagnification and bioaccumulation models for PFAS is in its infancy due to significant differences in uptake, excretion, and target organs/blood.
Another key chemical property used in ecological risk assessments that apply in an aquatic setting is the biota-sediment accumulation factor (BSAF). This factor is the ratio of the concentration of PFAS in biota to that of the sediment. Studies have been initiated to collect BSAF data to build a database. This is important because PFAS bioaccumulation is different from traditional chemicals of concern due to different transport mechanisms in the body (e.g., diffusion into/from lipid tissue vs protein binding and transport).
Draft Aquatic Life Criteria
On May 3, 2022, the United States Environmental Protection Agency (USEPA) published draft Recommended Aquatic Life Ambient Water Quality Criteria for PFOA and PFOS under the Clean Water Act (Federal Register, May 2022). Table 1, below, is reproduced from the Federal Register. The potential release of PFAS from underlying contaminated sediment and NAPL will affect water column concentrations and meeting these criteria.
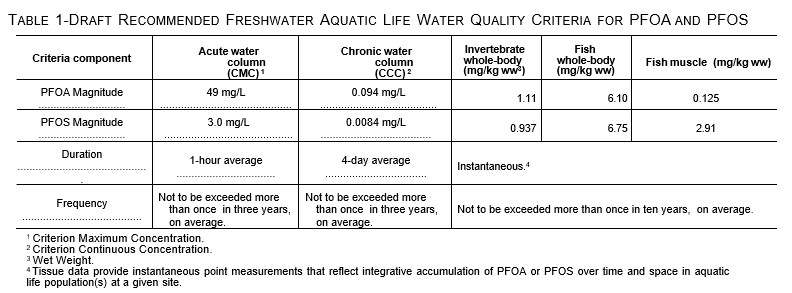
Remedial Considerations
For contaminated sediment sites, there are four primary technologies that are typically applied: monitored natural recovery (MNR), in-situ treatment, capping and dredging. For PFAS, MNR is anticipated to rely primarily on physical processes (e.g., burial by ongoing deposition of cleaner sediment from upstream sources) since many PFAS of current interest are highly resistant to degradation in the environment. Capping (Figure 2) can be implemented to replace the top of sediment to which biota is exposed and to retard migration of COCs from the underlying sediment into the water column. A cap may include a single layer or multiple layers comprising a chemical isolation layer, an erosion control layer and/or a habitat layer. The chemical isolation layer may be augmented with amendments such as organic carbon, activated carbon, or proprietary products such as Ziltech RembindTM, CETCO Fluoro-Sorb® or an engineered geomat that adsorbs chemicals like PFAS and thereby retard its movement through the cap.
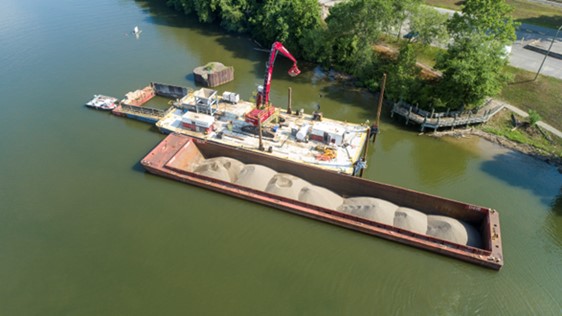
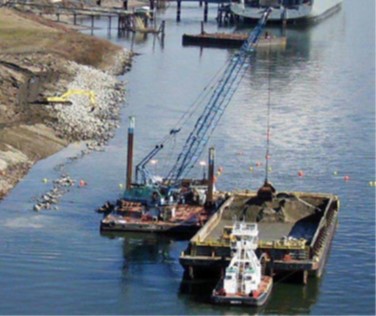
A Word of Caution
References
Ground (FTG), Distribution and Potential Future Release. J. Hazard. Mater., 296, 46-53.
Casado‐Martinez, C., Pascariello, S., Polesello, S., Valsecchi, S., Babut, M., Ferrari, B. J. D. (2021). Sediment Quality Assessment Framework for Per‐ and Polyfluoroalkyl Substances: Results From a Preparatory Study and Regulatory Implications. Integr. Environ. Assess. Manag., 17, (4), 716–725.
D’Ambro, E. L., Pye, H. O. T., Bash, J. O., Bowyer, J., Allen, C., Efstathiou, C., Gilliam, R. C., Reynolds, L., Talgo, B., Murphy, B. N. (2021). Characterizing the Air Emissions, Transport, and Deposition of Per and Polyfluoroalkyl Substances from a Fluoropolymer Manufacturing Facility. Environ. Sci. Technol., 55,
862-870.
Federal Register /Vol. 87, No. 171 /Tuesday, September 6, 2022 / Proposed Rules 54415-54441. Designation of Perfluorooctanoic Acid (PFOA) and Perfluorooctanesulfonic Acid (PFOS) as CERCLA Hazardous Substances.
Federal Register /Vol. 87, No. 85 /Tuesday, May 3, 2022 /Notices 26199-26201. Draft Recommended Aquatic Life Ambient Water Quality Criteria for Perfluorooctanoic Acid (PFOA) and Perfluorooctane Sulfonic Acid (PFOS).
MDEQ (2018) Sediment PFAS Sampling Guidance, Michigan Department of Environmental Quality.
Oliver, D. P., Navarro, D. A., Baldock, J., Simpson, S. L., Kookana, R. S. (2020). Sorption Behaviour of Per- and Polyfluoroalkyl Substances (PFASs) as Affected by the Properties of Coastal Estuarine Sediments. Sci. Total Environ., 720, Article137263.
Pétré, M-A., Genereux, D. P., Koropeckyj-Cox, L., Knappe, D R.U., Duboscq, S., Gilmore, T. E., Hopkins, Z. R. (2021). Per- and Polyfluoroalkyl Substance (PFAS) Transport from Groundwater to Streams near a PFAS Manufacturing Facility in North Carolina, USA. Environ. Sci. Technol., 55, 5848−5856.
Sima, M. W., Jaffé, P. R. (2021), A Critical Review of Modeling Poly- and Perfluoroalkyl Substances (PFAS) in the Soil-water Environment. Science of the Total Environment, 757, 143793.
Sörengård, M., Kleja, D. B., Ahrens, L. (2019). “Stabilization and Solidification Remediation of Soil Contaminated With Poly- and Perfluoroalkyl Substances (PFASs).” J. Hazard. Mater., 367, 639-646.
USEPA (2022). https://www.epa.gov/pfas/pfas-explained (Accessed October 7, 2022).
Van Glubt, S., Brusseau, M. L. (2021). Contribution of Nonaqueous-Phase Liquids to the Retention and Transport of Per and Polyfluoroalkyl Substances (PFAS) in Porous Media. Environ. Sci. Technol., 55, 3706−3715.
Research Corner

Doctor of Philosophy
Colorado School of Mines
Abstract
Related Links
ANSR Archives
Coming Up
Announcements
Upcoming ITRC Training
- December 6: Optimizing Injection Strategies and In Situ Remediation Performance
Upcoming IPEC Training
- December 1: Collaborative In-Situ Design Criteria and Strategy for Maximum Application Success / Surfactant Enhanced Extraction (SEE) of VOC, Sorbed, and NAPL Phases Exposing Factors which Limit their Remediation